DIGESTIVE VACUOLE OF PLASMODIA
2.3 Mechanisms of resistance of parasite to current
drugs
Despite the almost universal occurrence of
chloroquine resistance, available evidence suggests that resistance arises as a
result of a decrease in drug concentration at the site of action, rather than
any change in the target of the drug itself. Chloroquine accumulation in the
acidic food vacuole of the malaria parasite might occur by passive diffusion
down the pH gradient (ion trapping), by import via an ATP-dependent transporter
(active uptake) or by binding to FP (CQ receptor). The CQ in the food vacuole
will be almost exclusively diprotonated. The plasmodial P-glycoproteinhomolog-1
(Pgh-1) or the Plasmodium falciparum resistance transporter (PfCRT)
are implicated in CQ resistance and might modulate quinoline uptake directly,
by transporting drugs in and out of the food vacuole, or indirectly, by
contributing to the generation of a pH or electrochemical gradient as shown in
Figure 2-5. It has long been acknowledged that the pHDV could be
important in the mechanism of CQ resistance: CQ-resistant (CQR) parasites
accumulate much less CQ than do their CQ-sensitive (CQS) counterparts (Bray et
al., 1998). Following from the weak-base theory, small increases in
pHDV (in the order of 0.2-0.5 pH unit) would substantially reduce
the vacuolar concentration of CQ (Yayon et al., 1985; Foley and Tilley, 1998;
Dzekunov et al., 2000; Fidock et al., 2000; Hyde, 2002].
There is currently no evidence for clinically relevant
artemisinin resistance (Meshnick, 2002), but Kamchonwongpaison and co-workers
have reported that P. falciparum parasites in - thalassemic red cells
are more resistant to artemisinin and artesunate than parasites in genetically
normal cell (Kamchonwongpaison et al., 1994). Because P. falciparum
infected thalassemic red cells have a decreased capacity to accumulate drug. In
fact, the thalassemics are a group of genetic diseases with defects in globin
chain synthesis in -thalassemic, there is decrease in -globin chain whereas in
-thalassemia, there is a decrease in -globin synthesis. This result suggests
that special precaution should be made for using artemisinin compounds in the
treatment of malaria in any area where abnormal globin-gene frequency is high
since this will facilitate the development of artemisinin resistance.
Paradoxically, thalassemia appears to confer resistance to malaria infection in
vitro and in vivo (Weatherall, 1987; Yuthavong and Wilairat, 1993).
Figure 2-5 Possible mechanisms for
chloroquine (CQ) uptake and resistance. [Macreadie et al., 2000]
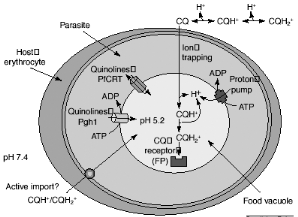
2.4 New strategy in the war against malaria
2.4.1 Discovering Antimalarials: New drug targets
The positive development over the last decade has been the
considerable increase in the understanding of processes occurring within the
parasite that are relevant to the mode of action of current antimalarials. It
also provides knowledge on targets or potential targets for new antimalarial
compounds.
It has been established that proteolysis of
hemoglobin is considered to be carried out by four aspartic proteases, namely
plasmepsins I, II, and IV, and histo-aspartic protease (HAP) (Banerjee, 2002),
three cysteine proteases (falcipains) (Rosenthal et al., 2002), and zinc
protease (falcilysin) (Eggleson, 1999). All of these represent potential
targets for antimalarials (Figure 2-4) and are currently the subject of intense
investigation.
Digestion of hemoglobin releases heme into the food vacuole,
where it is oxidized to hematin. Heme is another possible drug target and has
been implicated in the mode of action of endoperoxide antimalarials, such as
artemisinin and its derivatives (Robert et al., 2002).
Hematin is believed to be the target of chloroquine and other
quinoline antimalarials (Figure 2-4). It has been demonstrated that
histidine-rich protein 2 (HRP-2) has been implicated as an enzyme or, more
likely an initiator in the process of formation of hemozoin. Drugs such as
chloroquine have been proposed to inhibit hemozoin formation via direct
interaction with hematin (Kaschula et al., 2002), by displacing hematin from
HRP-2 (Pandey et al., 2001), or by preventing its binding to this protein.
HRP-2 represents a hitherto unexplored target for new antimalarials (Figure
2-4). In this issue of chemistry and biology, the development of a new
rapid-throughput screening method for investigating the ability of compounds to
prevent hematin binding to HRP-2 is reported recently (Choi et al., 2002;
Kannan et al., 2002).
These discoveries can be elaborated on in the future to yield
new drugs for treatment of this devastating disease (Egan, 2002).
|